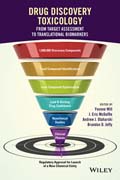
Drug Discovery Toxicology: From Target Assessment to Translational Biomarkers
Will, Yvonne
McDuffie, J. Eric
Olaharski, Andrew J.
Jeffy, Brandon D.
As a guide for pharmaceutical professionals to the issues and practices of drug discovery toxicology, this book integrates and reviews the strategy and application of tools and methods at each step of the drug discovery process. Guides researchers as to what drug safety experiments are both practical and useful Covers a variety of key topics safety lead optimization, in vitro–in vivo translation, organ toxicology, ADME, animal models, biomarkers, and omics tools Describes what experiments are possible and useful and offers a view into the future, indicating key areas to watch for new predictive methods Features contributions from firsthand industry experience, giving readers insight into the strategy and execution of predictive toxicology practices INDICE: PREFACE Eric Blomme .PART I. INTRODUCTION .1. EMERGING TECHNOLOGIES AND THEIR ROLE IN REGULATORY REVIEW Thomas Colatsky .1.1 INTRODUCTION .1.2 Safety Assessment in Drug Development and Review .1.2.1 Drug discovery .1.2.2 Preclinical development .1.3 The Role of New Technologies in Regulatory Safety Assessment .1.3.1 In silico models for toxicity prediction .1.3.2 Cell–Based Assays for Toxicity Prediction .1.4 CONCLUSIONS .REFERENCES .PART II. SAFETY LEAD OPTIMIZATION STRATEGIES .2. Small Molecule Safety Lead Optimization Donna Dambach .2.1 Background and Objectives of Safety Lead Optimization Approaches .2.2 Target Safety Assessments: Evaluation of Undesired Pharmacology and Therapeutic Area Consideration .2.3 Implementing Lead Optimization Strategies for Small Molecules .2.3.1 Strategic Approach .2.3.2 Application of Prospective Models .2.3.3 Selectivity and Secondary Pharmacology Assessments .2.3.4 Intrinsic Cytotoxicity Assessments .2.3.5 Focused Target Organ Assessments .2.3.6 ADME Assessments Related to Toxicity .2.3.7 Genotoxicity Assessments .2.3.8 Application of Retrospective Models .2.4 Conclusions .References .3. Safety Assessment Strategies and Predictive Safety of Biopharmacueticals and Antibody Drug Conjugates Michelle Horner, Mary–Jane Hinrichs, Nicholas Buss .3.1 Background and objectives .3.2 Target Safety Assessments: Strategies to Understand Target Biology and Associated Liabilities .3.3 Strategic Approaches for Biopharmaceuticals and Antibody Drug Conjugates (ADCs) .3.4 Predictive Safety Tools for large molecules .3.5 Predicting and Assessing unintended adverse consequences .3.6 Strategies for Species Selection .3.7 Strategy for Dose–ranging studies for safety evaluation of biopharmaceuticals .3.8 Conclusions .References .4. Discovery and Development Strategies for Small Interfering RNAs Scott Barros, Gregory Hinkle .4.1 Background .4.2 Target Assessments .4.3 siRNA Design and Screening Strategies .4.4 Safety Lead Optimization of siRNA .4.5 Integration of Lead Optimization Data for Candidate Selection and Development .4.6 Conclusions .References .PART III. BASIS FOR IN VITRO – IN VIVO PK TRANSLATION .5. Physicochemistry and the off target effects of drug molecules Dennis Smith .5.1 Lipohilicity, polar surface area and lipoidal permeability .5.2 Physicochemistry and basic ADME properties for high lipoidal permeability drugs .5.3 Relationship between Volume of Distribution (Vd) and Target Access for passively distributed drugs .5.4 Basicity, lipophilicity and volume of distribution as a predictor of toxicity (T): adding the T to ADMET .5.5 Basicity and lipophilicity as a predictor of toxicity (T): separating the D from T in ADMET .5.6 Lipophilicity and polar surface area as a predictor of toxicity): adding the T to ADMET .5.7 Metabolism and physicochemical properties .5.8 Concentration of compounds by transporters .5.9 Inhibition of excretion pumps .5.10 Conclusion .References .6. The need for exposure projection in the interpretation of preclinical in vitro and in vivo ADME tox data Patrick Poulin .6.1 Introduction .6.2 Methodology used for human PK projection in drug discovery .6.2.1 Prediction of plasma concentration–time profile by using the Wajima allometric method .6.2.2 Prediction of plasma and tissue concentration–time profiles by using the PBPK modeling approach .6.2.3 Integrative approaches of toxicity prediction based on the extent of tissue distribution .6.3 Summary of the take–home messages from the PhRMA CPCDC Initiative on Predictive Models of Human PK from 2011 .6.3.1 PhRMA initiative on the prediction of clearance .6.3.2 PhRMA initiative on the prediction of volume of distribution .6.3.3 PhRMA initiative on the prediction of concentration–time profile .6.3.4 Lead commentaries on the PhRMA initiative .References .7. ADME properties leading to toxicity Katya Tsaioun .7.1. Introduction .7.2. The Science of ADME .7.3. The ADME Optimization Strategy .7.4. Conclusions and Future Directions .References .PART IV. PREDICTING ORGAN TOXICITY .8. Liver Gerry Kenna, Mikael Persson, Scott Siler, Ke Yu, Weida Tong, Joshua Xu, Minjun Chen, Chuchu Hu, Yvonne Will, Mike Aleo .8.1. Introduction .8.2. DILI mechanisms and susceptibility .8.3. Common Mechanisms that contribute to DILI .8.3.1 Mitochondrial injury .8.3.2 Reactive metabolite mediated toxicity .8.3.3 Bile Salt Export Pump (BSEP) inhibition .8.3.4 Complicity between dual inhibitors of BSEP and mitochondrial function .8.4. Models Systems Used to Study DILI .8.4.1 High content image analysis .8.4.2 Complex cell models .8.4.3 Zebrafish .8.5. In Silico Models .8.6. Systems pharmacology and DILI .8.7. Summary .References .9. Cardiac David Gallacher, Robert Hamlin, Gary Gintant, Hugo Vargas, Kimberly Hoagland, Najah Abi–Gergis, HR Lu .9.1 General Introduction .9.2 Classical In Vitro/Ex Vivo Assessment of Cardiac Electrophysiologic Effects .9.2.1 Introduction .9.2.2 Subcellular techniques .9.2.3 Ionic currents .9.2.4 Action potentials/Repolarization assays .9.2.5 Proarrhythmia assays .9.2.6 Future directions .9.2.7 Conclusions .9.3 Cardiac ion channels and in silico prediction .9.4 From Animal Ex–vivo/in vitro models to Human Stem Cell–Derived Cardiomyocytes for Cardiac Safety Testing .9.4.1 Introduction .9.4.2 Currently available technologies .9.4.3 Conclusions .9.5 In Vivo Telemetry Capabilities and Preclinical Drug Development .9.6 Assessment of myocardial contractility in preclinical models .9.7 Assessment of large versus small molecules in cardiovascular safety pharmacology .9.8 Patients do not necessarily respond to drugs and devices as do genetically–identical, young–mature, healthy mice! .10. Predictive In Vitro Models for Assessment of Nephrotoxicity and Drug–Drug Interactions in Vitro Lawrence Lash .10.1. Introduction .10.1.1 Considerations for studying the kidneys as a target organ for drugs and toxic chemicals .10.1.2 Advantages and limitations of in vitro models in general for mechanistic toxicology and screening of potential adverse effects .10.1.3 Types of in vitro models available for studying human kidneys .10.2 Biological processes and toxic responses of the kidneys that are normally measured in toxicology research and drug development studies .10.3 Primary cultures of human proximal tubular (hPT) cells .10.3.1 Methods for hPT cell isolation .10.3.2 Validation of hPT primary cell cultures .10.3.3 Advantages and limitations of hPT primary cell cultures .10.3.4 Genetic polymorphisms and interindividual susceptibility .10.4 Toxicology studies in hPT primary cell cultures .10.5 Critical studies for drug discovery in hPT primary cell cultures .10.5.1 Phase I and Phase II drug metabolism .10.5.2. Membrane transport .10.6 Summary and conclusions .10.6.1 Advantages and limitations of performing studies in hPT primary cell cultures .10.6.2 Future Directions .REFERENCES .11. Predicting organ toxicity in vitro: Bone marrow Ivan Rich, Andrew Olaharski .11.1 Introduction .11.2 Biology of the hematopoietic system .11.3 Hemotoxicity .11.4 Measuring hemotoxicity .11.5 Proliferation or differentiation? .11.6 Measuring and Predicting Hemotoxicity in vitro .11.7 Detecting Stem and Progenitor Cell Downstream Events .11.8 Bone marrow toxicity testing during drug development .11.9 Predicting Starting Doses for Animal and Human Clinical Trials .11.10 Future Trends .11.11 Conclusions .References .12. Predicting Organ Toxicity In Vitro: Dermal Toxicity Patrick Hayden, Michael Bachelor .12.1 Introduction .12.2. Overview of drug–induced adverse cutaneous reactions .12.3. Overview of In Vitro skin models with relevance to preclinical drug development .12.4 Specific applications of in vitro skin models and predictive in vitro assays relevant to pharmaceutical development .12.4.1 Skin sensitization .12.4.2 phototoxicity .12.4.3 Skin irritation .12.5 Mechanism based cutaneous adverse effects .12.5.1 Percutaneous absorption .12.5.2 Genotoxicity .12.5.3 Skin Lightening/melanogenesis .12.6. Summary .References .13. In Vitro Methods in Immunotoxicity Assessment Xu Zhu, Ellen Evans .13.1. Introduction and Perspectives on In Vitro Immunotoxicity Screening .13.2. Overview of the Immune System .13.3. Examples of In Vitro Approaches .13.3.1 Acquired Immune Responses .13.3.2 Fc Receptor/Complement Binding .13.3.3 Assessment of Hypersensitivity .13.3.4 Immunogenicity of Biologics .13.3.5 Immunotoxicity Due to Myelotoxicity .13.4 Conclusions .References .14. Strategies and assays for minimizing risk of ocular toxicity during early development of systemically administered drugs Chris Somps, Jay Forner, Kerri Cannon, Wenhu Huang, Paul Butler .14.1. Introduction .14.2. In silico and In vitro tools and strategies .14.3. Higher throughput in vivo tools and strategies .14.3.1. Ocular reflexes and associated behaviors .14.3.2 Routine eye examinations .14.4. Strategies, gaps and emerging technologies .14.4.1 Strategic deployment of in silico, in vitro, and in vivo tools .14.4.2 Emerging biomarkers of retinal toxicity .14.5. Summary .References .15. Predicting organ toxicity in vitro – Central Nervous System Greet Teuns, Alison Easter .15.1. Introduction .15.2. Models for assessment of CNS ADRs .15.2.1. In vivo behavioral batteries .15.2.2. In vitro models .15.3. Seizure Liability Testing .15.3.1. Introduction .15.3.2. Medium/high throughput approaches to assess seizure liability of drug candidates .15.3.3. In vivo approaches to assess seizure liability of drug candidates .15.4. Drug Abuse Liability Testing .15.4.1. Introduction .15.4.2. Preclinical models to test abuse potential of CNS–active drug candidates .15.5. General Conclusions .References .16. Biomarkers, Cell Models and In Vitro Assays for Gastrointestinal Toxicology Gina Yanochko, Allison Vitsky .16.1. Introduction .16.2. Anatomic and physiologic considerations .16.2.1 Oral Cavity .16.2.2 Esophagus .16.2.3 Stomach .16.2.4 Small and large intestine .16.3. GI Biomarkers .16.3.1 Biomarkers of epithelial mass, intestinal function, or cellular damage .16.3.2 Biomarkers of inflammation .16.4. Cell Models of the GI Tract .16.4.1 Cell lines and primary cells .16.4.2 Induced pluripotent stem cells .16.4.3 Co–culture systems .16.4.4 3–D organoid models .16.4.5 Organs–on–a–chip .16.5. Cell–Based In Vitro Assays for Screening & Mechanistic Investigations to GI Toxicity .16.5.1 Cell viability .16.5.2 Cell migration .16.5.3 Barrier integrity .16.6. Summary, conclusions, and challenges .References .17. Preclinical Safety assessment of Drug Candidate–Induced pancreatic toxicity Karrie Brenneman, Shashi Ramaiah, Lauren Gauthier .17.1 Drug–Induced Pancreatic Toxicity .17.1.1 Introduction .17.1.2 Drug–Induced Pancreatic Exocrine Toxicity in Humans Pancreatitis .17.1.3 Mechanisms of Drug–Induced Pancreatic Toxicity .17.2. Preclinical Evaluation of Pancreatic Toxicity .17.2.1 Introduction .17.2.2 Risk Management and Understanding the Potential for Clinical Translation .17.2.3 Interspecies and Interstrain Differences in Susceptibility to Pancreatic Toxicity .17.3. Preclinical Pancreatic Toxicity Assessment – In Vivo .17.3.1 Routine Assessment .17.3.2 Specialized Techniques .17.4. Pancreatic Biomarkers .17.4.1 Introduction .17.4.2 Exocrine Injury Biomarkers in Humans and Preclinical Species .17.4.3 Endocrine/islet functional biomarkers for humans and preclinical species .17.4.4 A note on biomarkers of vascular injury relevant to the pancreas .17.4.5 Author s opinion on the strategy for investments to address pancreatic biomarker gaps .17.5. Preclinical Pancreatic Toxicity Assessment In Vitro .17.5.1 Introduction to Pancreatic Cell Culture .17.5.2 Modeling In Vivo Toxicity In Vitro, Testing Translatability and In Vitro Screening Tools .17.5.3 Case Study 1: Direct Acinar Cell Toxicant .17.5.4 Case Study 2: Primary Microvascular Toxicity with Secondary Endocrine/Exocrine Injury .17.5.5 Emerging Technologies/Gaps: Organotypic Models .17.6. Summary and Conclusions .References .PART V. ADDRESSING THE FALSE NEGATIVE SPACE– INCREASING PREDICTIVITY .18. Animal models of disease for future toxicity predictions Sherry Morgan and Chandikumar Elangbam .18.1 Introduction .18.2 HEPATIC DISEASE MODELS .18.2.1 Hepatic toxicity relevance to drug attrition .18.2.2 Hepatic toxicity Reasons for poor translation from animal to human .18.2.3 Available hepatic models to predict hepatic toxicity or understand molecular mechanisms of toxicity advantages and limitations .18.3 CARDIOVASCULAR DISEASE MODELS .18.3.1 Cardiac toxicity relevance to drug attrition .18.3.2 Cardiac toxicity Reasons for poor translation from animal to human .18.3.3 Available CV models to predict cardiac toxicity or understand molecular mechanisms of toxicity advantages and limitations .18.4 NERVOUS SYSTEM DISEASE MODELS .18.4.1 Nervous system toxicity relevance to drug attrition .18.4.2. Nervous system toxicity Reasons for poor translation from animal to human .18.4.3 Available nervous system models to predict nervous system toxicity or understand molecular mechanisms of toxicity advantages and limitations .18.5 GASTROINTESTINAL INJURY MODELS .18.5.1 Gastrointestinal (GIT) toxicity relevance to drug attrition .18.5.2 Gastrointestinal toxicity Reasons for poor translation from animal to human .18.5.3. Available gastrointestinal animal models to predict gastrointestinal toxicity or understand molecular mechanisms of toxicity advantages and limitations .18.6 RENAL INJURY MODELS .18.6.1. Renal toxicity relevance to drug attrition .18.6.2. Renal toxicity Reasons for poor translation from animal to human .18.6.3. Available Renal models to predict renal toxicity or understand molecular mechanisms of toxicity advantages and limitations .18.7 RESPIRATORY DISEASE MODELS .18.7.1. Respiratory toxicity relevance to drug attrition .18.7.2. Respiratory toxicity Reasons for adequate translation from animal to human .18.7.3. Available hepatic models to predict respiratory toxicity or understand molecular mechanisms of toxicity advantages and limitations .18.8 Conclusion .References .19. The Use of Genetically–Modified Animals in Discovery Toxicology Dolores Diaz and Jonathan Maher .19.1 Introduction .19.2 Use of Genetically Modified Animal Models in Discovery Toxicology .19.3 Use of Genetically Modified Animal Models in Pharmacokinetics and Metabolism .19.3.1 Drug Metabolism .19.3.2 Drug Transporters .19.3.3 Nuclear Receptors .19.3. 4 Humanized Liver Models .19.4. Conclusions .References .20. Addressing the False Negative Space– Increasing Predictivity Allison Harrill, Ted Choi .20.1. Introduction .20.2. Pharmacogenetics and Population Variability .20.3. Rodent Populations Enable a Population Based Approaches to Toxicology .20.3.1 Mouse Diversity Panel .20.3.2 Collaborative Cross Mice .20.3.3 Diversity Outbred Mice .20.4. Applications for Pharmaceutical Safety Science .20.4.1 Personalized Medicine Development of Companion Diagnostics .20.4.2 Biomarkers of Sensitivity .20.4.3 Mode of Action .20.5. Study Design Considerations for Genomic Mapping .20.5.1 Dose Selection .20.5.2 Model Selection .20.5.3 Sample Size .20.5.4 Phenotyping .20.5.5 Genome Wide Association Analysis .20.5.6 Candidate Gene Analysis .20.5.7 Cost Considerations .20.6. Summary .References .PART VI. STEM CELLS IN TOXICOLOGY .21. Application of pluripotent stem cells in drug–induced liver injury safety assessment Chris S. Pridgeon, Fang Zhang, James A. Heslop, Charlotte M.L. Nugues, Neil R. Kitteringham, B. Kevin Park, Chris E.P. Goldring .21.1 The liver, hepatocytes and drug–induced liver injury .21.2 Current models of DILI .21.2.1 Primary human hepatocytes .21.2.2 Murine models .21.2.3 Cell lines .21.2.4 Stem cell models .21.3 Uses of iPSC HLCs .21.4 Challenges of using IPSCs and new directions for improvement .21.4.1 Complex culture systems .21.4.2 Co–culture .21.4.3 3D culture .21.4.4 Perfusion bioreactors .21.5 Alternate uses of hepatocyte–like cells in toxicity assessment .References .22. Human pluripotent stem cell–derived cardiomyocytes Praveen Shukla, Priyanka Garg, Joseph Wu .22.1. Introduction .22.2. Advent of hPSCs: reprogramming and cardiac differentiation .22.2.1 Reprogramming .22.2.2 Cardiac differentiation .22.3. iPSC–based disease modeling and drug testing .22.4. Traditional target–centric drug discovery paradigm .22.5. iPSC–based drug discovery paradigm .22.5.1 Target identification and validation: clinical trial in a dish .22.5.2 Safety pharmacology and toxicological testing .22.6. Limitations & challenges .22.7. Conclusion and future perspective .References .23 Stem cell–derived renal cells and predictive renal in vitro models Jacqueline Kai, Yue Ning, Peng Huang, Daniel Zink .23.1. Introduction .23.2. Protocols for the differentiation of pluripotent stem cells into cells of the renal lineage .23.2.1. Earlier protocols and the recent race .23.2.2. Protocols designed to mimic embryonic kidney development .23.2.3. Rapid and efficient methods for the generation of proximal tubular–like cells .23.3. Renal in vitro models for drug safety screening .23.3.1. Microfluidic and 3D models and other models that have been tested with lower numbers of compounds .23.3.2. In vitro models that have been tested with higher numbers of compounds and the first predictive renal in vitro model .23.3.3. Stem cell–based predictive models .23.4. Achievements and future directions .Acknowledgements .References .PART VII. CURRENT STATUS OF PRECLINICAL IN VIVO TOXICITY BIOMARKERS .24 Predictive Cardiac Hypertrophy Biomarkers in Non clinical Studies Steven Engle .24.1. Introductory Background: Cardiovascular Toxicity .24.2. Cardiac Hypertrophy .24.3. Biomarkers of Cardiac Hypertrophy .24.4. Case Studies .24.5. Conclusion .References .25. Vascular Injury Biomarkers Tanya Zabka and Kaidre Bendjama .25.1. Historical context of drug–induced vascular injury and drug development .25.2. Current state of DIVI biomarkers .25.3. Current status and future of in vitro systems to investigate DIVI .25.4. Incorporation of in vitro and in vivo tools in preclinical drug development .25.5. DIVI Case Study .References .26. Novel Translational Biomarkers of Skeletal Muscle Injury Peter Burch and Warren Glaab .26.1. Introduction .26.2. Overview of drug–induced skeletal muscle injury .26.3.1 Skeletal Troponin I (sTnI) .26.3.2 Creatine Kinase M (CKM) .26.3.3 Myosin Light Chain 3 (Myl3) .26.3.4 Fatty Acid Binding Protein 3 .26.3.5 Parvalbumin .26.3.6 Myoglobin .26.3.7 MicroRNAs .26.4. Regulatory Endorsement .26.5. Gaps and Future directions .26.6. Conclusions .References .27. Translational Mechanistic Biomarkers and Model for Predicting Drug–Induced Liver Injury Daniel Antoine .27.1. Introduction .27.2. Drug–Induced Toxicity and the Liver .27.3. Current Status of Biomarkers for the Assessment of DILI .27.4. Novel Investigational Biomarkers for DILI .27.4.1. Glutamate Dehydrogenase (GLDH) .27.4.2. Acylcarnitines .27.4.3. High Mobility Group Box–1 (HMGB1) .27.4.4. Keratin–18 (K18) .27.4.5. MicroRNA–122 (miR–122) .27.5. In Vitro Models and the Prediction of Human DILI .27.6. Conclusions and Future Perspectives .References .PART VIII. Kidney Injury Biomarkers .28. Assessing and Predicting Drug–Induced Kidney Injury, Functional Change and Safety in Preclinical Studies in Rats Yafei Chen .28.1. Introduction .28.2. Kidney Functional Biomarkers (Glomerular Filtration and Tubular Reabsorption) .28.2.1. Traditional Functional Biomarkers .28.2.2. Novel Functional Biomarkers .28.3. Novel Kidney Tissue Injury Biomarkers .28.3.1. Urinary N–acetyl–beta–D–glucosaminidase (NAG) .28.3.2. Urinary Glutathione S–transferase alpha ( –GST) .28.3.3. Urinary Renal Papillary Antigen 1 (RPA–1) .28.3.4. Urinary Calbindin D28 .28.4. Novel Biomarkers of Kidney Tissue Stress Response .28.4.1. Urinary Kidney Injury Molecule–1 (KIM–1) .28.4.2. Urinary Clusterin .28.4.3. Urinary Neutrophil Gelatinase–associated Lipocalin (NGAL) .28.4.4. Urinary Osteopontin (OPN) .28.4.5. Urinary L–type Fatty Acid Binding Protein (L–FABP) .28.4.6. Urinary Interleukin 18 (IL–18) .28.5. Application of an Integrated Rat Platform (Automated Blood Sampling and Telemetry; ABST) for Kidney Function and Injury Assessment .References .29. Canine Kidney Safety Protein Biomarkers Manisha Sonee .29.1. Introduction .29.2. Novel Canine Renal Protein Biomarkers .29.3. Evaluations of Novel Canine Renal Protein Biomarker Performance .29.4. Conclusion .References .30. Traditional Kidney Safety Protein Biomarkers and Next–Generation Drug–Induced Kidney Injury Biomarkers in Nonhuman Primates Jean–Charles Gautier and Xiaobing Zhou .30.1. Introduction .30.2. Evaluations of Novel Nonhuman Primate Renal Protein Biomarker Performance .30.3. New Horizons: Urinary MicroRNAs and Nephrotoxicity in NHPs .References .31. Rat Kidney MicroRNA Atlas Aaron Smith .31.1 Introduction .31.2 Key findings .References .32. MicroRNAs as Next–Generation Kidney Tubular Injury Biomarkers in Rats Heidrun Ellinger–Ziegelbauer and Rounak Nassirpour .32.1. Introduction .32. 2. Rat Tubular MicroRNAs .32.3. Conclusions .References .33. MicroRNAs as Novel Glomerular Injury Biomarkers in Rats Rachel Church .33.1. Introduction .33.2. Rat Glomerular MicroRNAs .References .34. Integrating Novel Imaging Technologies to Investigate Drug–Induced Kidney Toxicity Bettina Wilm and Neal Burton .34.1. Introduction .34.2. Overview .34.3. Summary .References .35. In vitro–to–In Vivo Relationships with Respect to Kidney Safety Biomarkers Paul Jennings .35.1 Renal cell lines as tools for toxicological investigations .35.2. Mechanistic approaches and in vitro to in vivo translation .35.3. Closing Remarks .References .36. Case Study: Fully Automated Image Analysis of Podocyte Injury Biomarker Expression in Rats Jing Ying Ma .36.1. Introduction .36.2. Material and Methods .36.3. Results .36.4. Conclusions .References .37. Case Study: Novel Renal Biomarkers translation to humans Deborah Burt .37.1. Introduction .37.2. Implementation of Translational Renal Biomarkers in Drug Development .37.3. Conclusion .References .38. Case Study: MicroRNAs as Novel Kidney Injury Biomarkers in Canines Craig Fisher, Erik Koenig, and Patrick Kirby .38.1 Introduction .38.2. Material and Methods .38.3. Results .38.4. Conclusions .References .39. Novel Testicular Injury Biomarkers Hungyun Lin .39.1. Introduction .39.2. The Testis .39.3. Potential Biomarkers for Testicular Toxicity .39.3.1. Inhibin B .39.3.2. Androgen–Binding Protein .39.3.3. SP22 .39.3.4. Emerging Novel Approaches .39.4. Conclusions .References .PART IX. Best Practices in Biomarker Evaluations .40. Best Practices in Preclinical Biomarker Sample Collections Jacqueline Tarrant .40.1. Considerations for reducing preanalytical variability in biomarker testing .40.2. Biological sample matrix variables .40.3. Collection variables .40.4. Sample processing and storage variables .References .41. Best Practices in Novel Biomarker Assay Fit–for–Purpose Testing Karen Lynch .41.1. Introduction .41.2. Why Use a Fit–for–Purpose Assay? .41.3. Overview of Fit–for–Purpose Assay Method Validations .41.4. Assay Method Suitability in Preclinical Studies .41.5. Best Practices for Analytical Methods Validation .41.5.1. Assay Precision .41.5.2. Accuracy/Recovery .41.5.3. Precision and Accuracy of the Calibration Curve .41.5.4. Lower Limit of Quantification .41.5.5. Upper Limit of Quantification .41.5.6. Limit of Detection .41.5.7. Precision Assessment for Biological Samples .41.5.8. Dilutional Linearity and Parallelism .41.5.9. Quality Control .41.6. Species– and Gender–Specific Reference Ranges .41.7. Analyte Stability .41.8. Additional Method Performance Evaluations .References .42. Best Practices in Evaluating Novel Biomarker Fit–for–Purpose and Translatability Amanda Baker .42.1. Introduction .42.2. Protocol Development .42.3. Assembling an Operations Team .42.4. Translatable Biomarker Use .42.5. Assay Selection .42.6. Biological Matrix Selection .42.7. Documentation of Patient Factors .42.8. Human Sample Collection Procedures .42.8.1. Biomarkers in Human Tissue Biopsy and Biofluid Samples .42.9. Choice of Collection Device .42.9.1. Tissue Collection Device .42.9.2. Plasma Collection Device .42.9.3. Serum Collection Device .42.9.4. Urine Collection Device .42.10. Schedule of Collections .42.11. Human Sample Quality Assurance .42.11.1. Monitoring Compliance to Sample Collection Procedures .42.11.2. Documenting Time and Temperature from Sample Collection to Processing .42.11.3. Optimal Handling and Preservation Methods .42.11.4. Choice of Sample Storage Tubes .42.11.5. Choice of Sample Labeling .42.11.6. Optimal Sample Storage Conditions .42.12. Logistics Plan .42.13. Database Considerations .42.14. Conclusive Remarks .References .43. Best Practices in Translational Biomarker Data Analysis Robin Mogg and Daniel Holder .43.1. Introduction .43.2. Statistical Considerations for Pre–Clinical Studies of Safety Biomarkers .43.3. Statistical Considerations for Exploratory Clinical Studies of Translational Safety Biomarkers .43.4. Statistical Considerations for Confirmatory Clinical Studies of Translational Safety Biomarkers .43.5. Summary .References .44 Translatable Biomarkers in Drug Development John–Michael Sauer, Elizabeth G Walker, and Amy C Porter .44.1. Safety Biomarkers .44.2. Qualification of Safety Biomarkers .44.3. Letter of Support for Safety Biomarkers .44.4. Critical Path Institute s Predictive Safety Testing Consortium .44.5. Predictive Safety Testing Consortium and its Key Collaborations .44.6. Advancing the Qualification Process and Defining Evidentiary Standards .References .PART X CONCLUSIONS .45 Toxicogenomics in Drug Discovery Toxicology Brandon Jeffy, Richard Brennan, Joseph Milano .45.1 A Brief History of Toxicogenomics .45.2 Tools and strategies for analyzing Toxicogenomics Data .45.3 Drug Discovery Toxicology Case Studies .References .46 Issue Investigation and Practices in Discovery Toxicology Dolores Diaz, Dylan Hartley Ray Kemper .46.1 Introduction .46.2 Overview of Issue Investigation in the Discovery Space .46.3 Strategies to Address Toxicities the Discovery Space .46.4 Cross–functional collaborative model .46.5 Case–Studies of Issue Resolution in the Discovery Space .46.6 Data inclusion in Regulatory Filings .References .Concluding Remarks Yvonne Will, Eric McDuffie, Andrew Olaharski and Brandon Jeffy .Index
- ISBN: 978-1-119-05333-0
- Editorial: Wiley–Blackwell
- Encuadernacion: Tela
- Páginas: 584
- Fecha Publicación: 13/05/2016
- Nº Volúmenes: 1
- Idioma: Inglés